
Profile: Clean hydrogen on demand
The Laboratory of Physical and Analytical Electrochemistry explain their innovative battery concept. By generating hydrogen, their redox flow battery offers new opportunities in renewable energy storage.
The growing production of renewable energy by wind turbines and photovoltaic panels and its progressive integration in the centralised grid have created new energy management issues due to the intermittent and unpredictable nature of these production means. A restructuring of the electrical grid is therefore necessary to ensure that electricity is reliably delivered to consumers. Energy storage is critical in order to compensate for the imbalance between consumers and producers. The fragmentation of the grid into more local, self-sustainable electricity networks – comprising both producers and consumers of electricity – also requires the installation of energy storage units to provide a stable electricity supply. Indeed, as power networks grow smaller, the need for energy storage increases.
Batteries for the electrical grid
Energy storage systems may be broadly categorised according to the amount of time energy will be stored for: long term (days), intermediate (hours), and short term (seconds to minutes). The intermediate and long term energy storage technologies are currently limited to hydropower and compressed air energy storage, which are practically restricted to specific geographical locations (e.g. existing lakes/reservoirs and underground caverns).
In the last ten years, advances in megawatt-scale batteries have thrust these technologies to the foreground of the field, and demonstration projects for renewable energy storage continue to emerge. Batteries provide major advantages such as flexibility in terms of location, capacity and power scales. Their characteristic fast response time (typically less than a second for starting a charge or discharge), low maintenance requirements and reliability favour their use for intermediate scale energy storage, particularly in the case of a delocalised electricity network. For instance, they are suitable for storing the electricity needed for a group of houses or an apartment building during the peak demand periods when the production is insufficient for supplying the required energy.
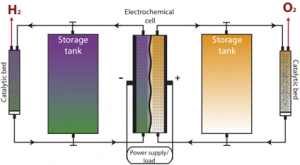
Concept of an external chemical discharge of an RFB: the charged liquids stored in the tanks can be discharged in the catalytic bed to generate hydrogen and oxygen, for instance
Redox flow batteries
In the large family of batteries, redox flow batteries (RFB) are particularly interesting for the hour-range storage of renewable energy. These batteries are based on two liquids which are pumped through a central electrochemical cell – where the energy is stored/released – and stored in separate, external reservoirs. The advantage of this approach compared to classical solid-state batteries is the independence between the energy and power ratings, which is widening the range of applications. As a result, these systems may be included in a local grid for compensating for peak electricity demand, which requires high power for a short time, or they may be coupled with a wind turbine or a photovoltaic panel installation in order to buffer the power output. The latter application requires higher storage capacities, associated with a larger size of the battery, as the amount of liquid is directly proportional to the energy capacity of the battery. Indeed, the limited energy density of RFBs results in large volume systems when substantial amounts of energy need to be stored. Such large volumes are not always practical and may increase the cost of the system.
Parallel circuits
The present project was motivated by this need to improve the energy density of RFBs. The concept is based on the observation that the charged liquids of the battery could be discharged via a secondary chemical reaction to generate hydrogen, instead of being discharged conventionally to generate electricity (Fig. 1). The discharged liquids can then return into the RFB for further charging. The hydrogen gas produced can be compressed and stored for different uses, such as fuel for fuel cell vehicles, or be converted again into electricity in a stationary fuel cell. While one half of the battery is being chemically discharged to produce hydrogen, the other half can also be chemically discharged, but this reaction is more dependent on the chemistry of the RFB.
The unique aspect of this approach is the incorporation of a parallel circuit, which permits the production of secondary products. For hydrogen production, this secondary circuit is composed of a bed of inexpensive molybdenum-based catalyst. In the dual-circuit configuration, the reaction rate for hydrogen evolution is not required to be particularly fast, as in a conventional electrolyser. In this way, the amount of energy stored by this modified RFB is no longer limited by its size, and surplus renewable energy can continue to be stored.
Demonstration
Currently, in order to validate this concept a demonstrator based on a modified 10kW (40kWh) all-vanadium RFB is operating in Martigny, Switzerland (Fig. 2). A commercial RFB was acquired and equipped with the secondary circuit for hydrogen generation on demand. Typical profiles of energy production by wind turbines and a district electrical consumption can be applied to this modified RFB, and the secondary circuit can be used to generate hydrogen when the surplus energy exceeds the capacity of the battery.
The efficiency of the RFB is about 80%, depending on the power of charge and discharge. However, when the system is discharged through the production of hydrogen, its energy efficiency is lowered – the efficiency depends on the RFB chemistry and the chemical discharge reactions, which is not considered a limiting aspect of this system as the source of energy is renewable, and thus cheap or even free. To date, this concept has been tested on two specific chemistries of RFBs: all-vanadium and cerium-vanadium redox chemistries. However, it may be extended to other RFBs. The long term objective is to be able to apply it to any commercial RFB in the form of a plug-in system that can be added to increase their energy capacity.
Future projects for the demonstration site of Martigny are currently being developed. For instance, the installation of a hydrogen refuelling station based on the production of hydrogen by an electrolyser and using an RFB for the buffering of surplus energy is expected to start by the end of 2015.
Professor Hubert Girault
Laboratory of Physical and Analytical Electrochemistry
Energypolis
EPFL
tel: +41 21 69 33151